Transport mechanism of doxorubicin loaded Chitosan based nanogels across intestinal epithelium
Authors
Chao Feng a, Guohui Sun a, Zhiguo Wang b, Xiaojie Cheng a, Hyunjin Park c, Dongsu Cha c, Ming Kong a,*,1, Xiguang Chen a,*,1 (2014)
a. College of Marine Life Science, Ocean University of China, Qingdao, China
b. Department of Plastic Surgery, The Affiliated Hospital of Medical College Qingdao University, Qingdao, China
c. Graduate School Biotechnology, Korea University, Seoul, South Korea
Introduction
Anticancer drugs, administered through i.v. injection or infusion during chemotherapy entails numerous side effects due to the direct delivery of high concentration anticancer-drug to bloodstream. These inconveniences compromise the clinical treatment efficacy. Unfortunately, most anticancer drugs especially those with excellent anticancer effects such as ; doxorubicin hydrochloride (DOX) and Taxanes (paclitaxel and docetaxel), are not orally bioavailable owing to inadequate transport throughout the intestinal epithelium. Recent studies demonstrated that Chitosan/carboxymethyl chitosan nanogels (CS/CMCS-NGs) could enhance the oral bioavailability of doxorubicin hydrochloride (DOX). Indeed, to improve oral bioavailability of anticancer drugs, CS/CMCS-NGs were successfully developed in our study. The rational design of these nanocarriers was to retain the promising behavior of CS as an absorption enhancer, yet expand the range of absorption enhancement from limited duodenal segment to the entire small intestine. To identify the mechanisms that support this recent observation, different transport pathways of CS/CMCS-NGs through the small intestine were studied in this work.
Objective
This study was to investigate the mechanism regarding the transport of CS/CMCS-NGs throughout the intestinal barrier, particularly focused on the cellular and molecular mechanisms. Finally, the transport mechanism of this nanocarrier was evaluated ex vivo and in vivo using animal models and confirmed the findings of in vitro studies (using Caco-2 cell line).
Material & methods
Preparation of Cy3-CS, FITC-CMCS and DOX:CS/CMCS-Ngs. Cy3-labeled chitosan (Cy3-CS) and FITC-labeled carboxymethyl chitosan (FITC-CMCS) were synthesized. The synthesis of Cy3-CS was based on the reaction between the free amines on the chitosan and N-hydroxy-succinimide on Cy3– SE. The synthesis of FITC-CMCS was based on the reaction between the amine groups of O-CMCS and the isothiocyanate group of FITC. DOX-loaded CS/CMCS-NGs (DOX:CS/CMCS-NGs) were also prepared. The capacity to bind Ca2+ of CS/CMCS-NGs was also investigated.
In vivo and ex vivo biodistribution. Overnight-fasted SD rats were used in the biodistribution study. DOX:CS/CMCSNGs or DOX:CS/CMCS-NGs with 20 mg/kg of CaCl2 dissolved in saline were orally administrated at a dose of 5 mg DOX-equiv./kg body weight to investigate the effect of Ca2+ on NGs-induced absorption enhancement. The rats were anesthetized with an injection of 10% chloral hydrate (0.004 mL/g); then, fluorescence images were obtained by small animal in vivo imaging instrument (Fusion FX7, Vilber Lourmat, France) at specific time intervals (0 h [untreated], 2 h, 4 h and 8 h). Filter sets (blue: excitation, 500– 720 nm; exposure time, 300 ms and red: excitation, 670–900 nm; exposure time, 150 ms) were used to detect DOX-related fluorescence. At 10 h after oral administration, major organs (intestine, stomach, heart, liver, spleen, lung, and kidneys) were dissected from SD rats’ bodies, then, observed by small animal in vivo imaging instrument (Fusion FX7, Vilber Lourmat, France).
Results
Figure 1. In vivo noninvasive fluorescence imaging DOX distribution in rats. The biodistribution of DOX:CS/CMCS-NGs was investigated in rats (Sprague–Dawley, 220–250 g) and the fluorescent signal of DOX was detected by the small animal in vivo imaging instrument (Fusion FX7, Vilber Lourmat, France). To confirm the transport mechanism of DOX:CS/CMCS NGs, high doses of the CaCl2 were orally co-administrated with DOX:CS/CMCS-NGs to weaken their capacity to binding Ca2+. Real-time images of DOX:CS/CMCS-NGs with or without the Ca2+ are presented in Fig. A. No apparent fluorescent signal was observed in the DOX:CS/CMCS-NGs with Ca2+ group during 8h detection. This may be due to the weakened capacity to binding Ca2+ of DOX:CS/CMCS-NGs being followed by a reduction in absorption DOX. Above in vivo results confirmed that the enhanced DOX absorption was determined by the capacity to binding Ca2+ of CS/ CMCS-NGs, which were consistent with the results of the in vitro transport studies.
Figure 2. Ex vivo fluorescence imaging of organs at 10 h post-oral administration. On the contrary, fluorescent intensity of DOX in DOX:CS/CMCS-NGs without Ca2+ group was strong for the first 4 h and maintained for 8 h. Furthermore, the major organs were dissected at 10 h after oral administration and observed by ex vivo imaging (Fusion FX7, Vilber Lourmat, France) (Fig. 2). Fluorescent signal in DOX:CS/CMCSNGs with Ca2+ group detected only in ileum and stomach. But for DOX : CS/CMCS-NGs without Ca2+ group, fluorescence signal was apparently rich in liver and kidneys, besides ileum and stomach.
Results
The results demonstrated that CS/CMCS-NGs had a strong binding ability of Ca2+. CS/CMCS-NGs could markedly enhance paracellular transport of DOX throughout the entire small intestine by chelating Ca2+. However, it has been demonstrated that the extra added Ca2+ weakened the chelating ability of CMCS and thus inhibited NGs-induced TJs opening. The high transport capacity of CS/CMCS-NGs by paracellular and transcellular pathway guaranteed the excellent absorption of encapsulated DOX throughout the entire small intestine.
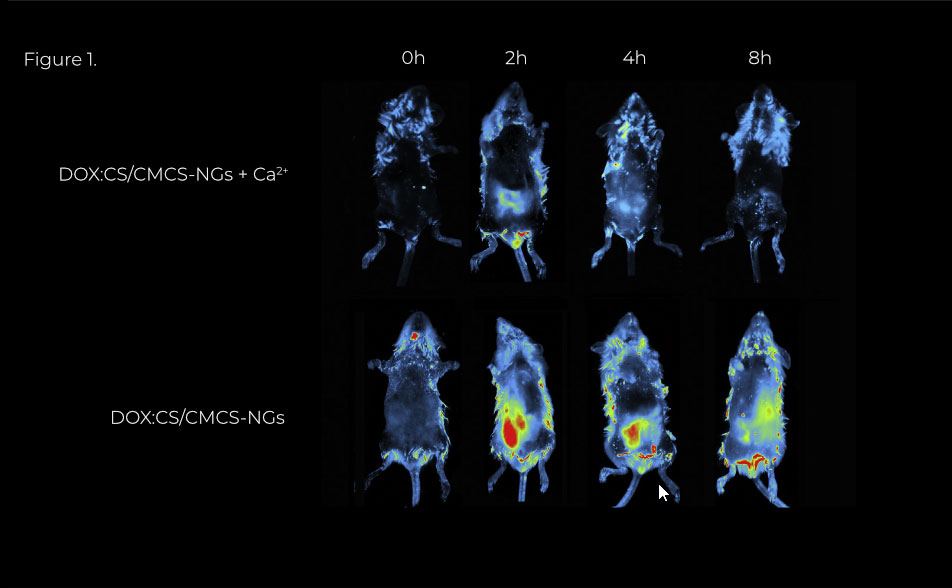
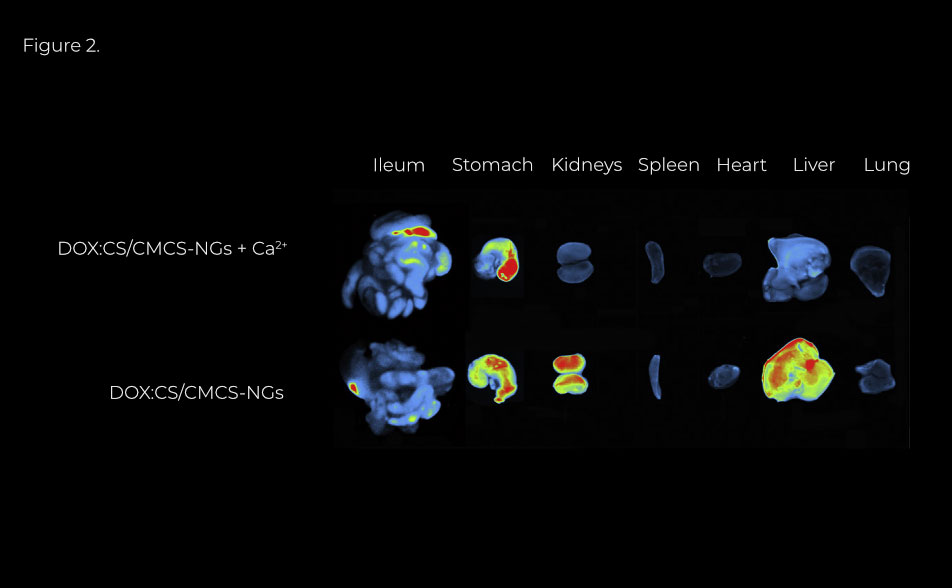